Research interests of the Lab
I. Mechanism of proofreading during translation of the genetic code : Structural basis of editing mechanism
Error-correction processes of the cell ensure quality control during protein synthesis. Aminoacyl-tRNA synthetases (aaRSs) establish the rules of the genetic code by attaching the correct amino acid to the cognate tRNA. The mechanism that selectively removes D-amino acids that are erroneously coupled to tRNAs and termed as “chiral proofreading” is performed by a dimeric enzyme, the D-amino acyl-tRNA deacylase (DTD) which has a key role in enforcing homochirality during translation.
- Mechanism of DTD in higher organisms in context of neuronal cells abundant in D-amino acids with high levels of DTD expression.
- in vivo studies on DTD knockdown and knockout lines in animal models such as Drosophila, zebrafish and mouse.
- Exploring other chiral proofreading enzymes such as DTD2 from archaea and plants, and DTD3 from certain cyanobacteria.
- Role of threonyl-tRNA synthetases (ThrRSs) from pathogenic organisms, with respect to amino acid recognition and editing mechanism.
|
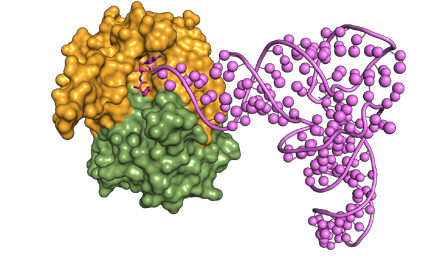
D-aminoacyl-tRNA captured by DTD for cleavage |
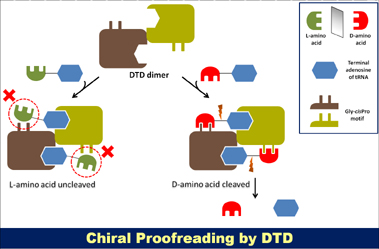
Schematic showing the crucial role of cross-subunit Gly-cisPro motif in providing chiral specificity to DTD dimer |
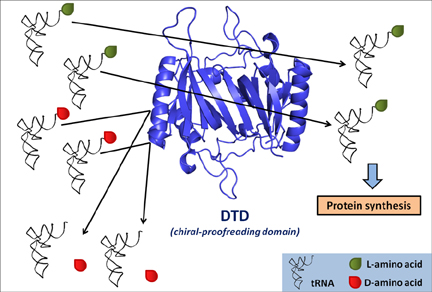
Chiral proofreading enzyme DTD selectively removes D-amino acids from tRNAs and ensures homochirality during translation
|
References:
- Distinct localization of chiral proofreaders resolves organellar translation conflict in plants.
Proc Natl Acad Sci U S A (2023) doi: 10.1073/pnas.2219292120.
- Design principles and functional basis of enantioselectivity of alanyl-tRNA synthetase and a chiral proofreader during protein biosynthesis.
Nucleic Acids Res. (2023) doi: 10.1093/nar/gkad205.
- Chiral Proofreading during protein biosynthesis and its evolutionary implications. FEBS Lett 2022; 596 DOI: 10.1002/1873-3468.14419
-
Switching a conflicted bacterial DTD-tRNA code is essential for the emergence of mitochondria.
Sci. Adv. 2022; 8, eabj7307, 1-9. doi: 10.1126/sciadv.abj7307.
- Recruitment of Archaeal DTD is a Key Event Toward the Emergence of Land Plants. Sci. Adv. 2021; 7 : eabe8890 DOI: 10.1126/sciadv.abe8890
- Genomic innovation of ATD alleviates mistranslation associated with multicellularity in Animalia. elife 2020;9:e58118.
(Covered by elife Science Digest- June 18 2020: 'Proofreading to evolve' )
- Chiral checkpoints during protein biosynthesis. J. Biol. Chem 2019; doi: 10.1074/jbc.REV119.008166
- Enzyme action at RNA-protein interface in DTD-like fold. Curr Opin Struct Biol. 2018; 53:107-114.
- A discriminator code–based DTD surveillance ensures faithful glycine delivery for protein biosynthesis in bacteria. elife 2018; doi: 10.7554/eLife.38232.
- A chiral selectivity relaxed paralog of DTD for proofreading tRNA mischarging in Animalia. Nature Commun. 2018; 9:511 DOI: 10.1038/s41467-017-02204-w
- Role of D-aminoacyl-tRNA deacylase beyond chiral proofreading as a cellular defense against glycine mischarging by AlaRS. elife 2017; doi: 10.7554 eLife.24001.
- Elongation factor Tu prevents misediting of Gly-tRNA(Gly) caused by the design behind the chiral proofreading site of D-aminoacyl-tRNA deacylase. PLOS Biol. 2016; 14:5 e1002465, 1-22.
- Specificity and catalysis hardwired at the RNA-protein interface in a translational proofreading enzyme. Nature Commun. 2015; 6:7552 doi: 10.1038/ncomms8552.
- Mechanism of chiral proofreading during translation of the genetic code. eLife (Cambridge). 2013; doi: 10.7554/eLife.01519
(highlighted by Science (343) Editor’s Choice, “Chirality check”, pp 119, 10th Jan, 2014).
- Mechanistic insights into cognate substrate discrimination during proofreading in translation. Proc. Natl. Acad. Sci. (USA); 2010, 107, 22117-22121.
- Posttransfer editing mechanism of a D-aminoacyl-tRNA deacylase-like domain in threonyl-tRNA synthetase from archaea. EMBO J.; 2006, 25, 4152-4162.
- A D-amino acid editing module coupled to the translational apparatus in archaea. Nature Struct. Mol. Biol.; 2005, 12, 556-557.
|
II. Structure-function relationship of lipid metabolizing enzymes in eukaryotes
Fatty acids are fundamental constituents of biological membranes,
energy generation processes, and are activated by a class of enzymes called Fatty acyl-CoA Ligases (FACL).
However, Fatty acyl-AMP ligases (FAAL), a structural homolog of FACLs, redirect the metabolic fate of fatty acids towards
secondary metabolite and complex lipid biosynthesis. Structural and biochemical studies in our lab have provided a mechanistic
basis for the metabolic dichotomy enforced by FAALs. Phylogenetic analysis across life forms has led to the identification of
FAAL-like domains in a eukaryotic supergroup called Opisthokonta (fungi and animalia).
Spatial segregation of different biological processes into membrane-bound organelles, essential for
carrying out vast array of complex cellular phenomena, is a characteristic of eukaryotic cells.
A defining feature of cellular compartmentalization is the “lipidome” of organellar membranes.
Our research identified a unique regulator of one such lipid, diacylglycerol (DAG), called disco-interacting protein 2 (DIP2),
which is conserved from yeast to mammals. DIP2 is a eukaryotic innovation, resulting from stitching two ancient FAAL-like domains
from bacteria together, repurposed to act on a chemically distinct subset of DAGs. DIP2 resides in mitochondria-vacuole contact sites
and is indispensable for maintaining organellar and thereby cellular homeostasis. We are currently interested in:
- Elucidating the structural and biochemical basis of DIP2’s function.
- Understanding DIP2’s role in organelle communication and physiology.
- Exploring the role of DIP2 homologs across eukaryotes using model systems such as Drosophila, mouse and human cell lines.
- Uncovering the evolutionary significance of recruiting FAAL-like domains in Opisthokonts.
- Understanding the role of DIP2 in pathogenic organisms.
Our research integrates biochemical, structural and cell biological approaches to unravel the roles of these ancient modules.
|
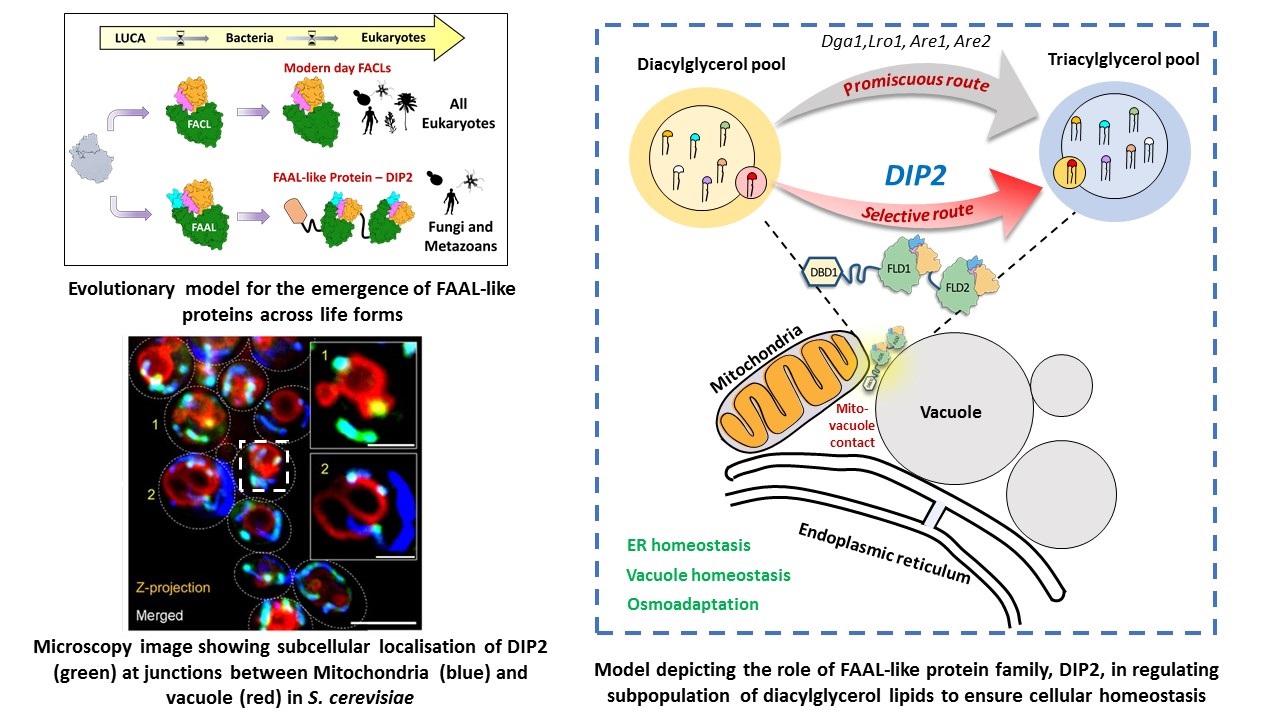
|
References:
- DIP2 is a unique regulator of diacylglycerol lipid homeostasis in eukaryotes. elife 2022;11:e77665.
- A universal pocket in fatty acyl-AMP ligases ensures redirection of fatty acid pool away from coenzyme A-based activation. elife elife 2021;10:e70067.
- Fatty acyl-AMP ligases as mechanistic variants of ANL superfamily and molecular determinants dictating substrate specificities. Journal of the Indian Institute of Science 2018; 98: 261-272
- Structural insights into the regulation of NADPH binding to reductase domains of nonribosomal peptide synthetases: A concerted loop movement model. J Struct. Biol. 2016; 194: 368-374.
- Unsaturated Lipid Assimilation by Mycobacteria Requires Auxiliary cis-trans Enoyl CoA Isomerase. Chemistry & Biology 2015; 22:1577-1587.
- Delineating the reaction mechanism of reductase domains of Nonribosomal Peptide Synthetases from mycobacteria.J. Struct. Biol. 2014; 187:207-214.
- Nonprocessive [2+2]e- Offloading Reductase Domains from Mycobacterial Non-ribosomal Peptide Synthetases. Proc. Natl. Acad. Sci. (USA); 2012, 109, 5681-5686.
- Molecular basis of the functional divergence of fatty acyl-AMP ligase biosynthetic enzymes of Mycobacterium tuberculosis. J. Mol. Biol.; 2012, 416, 221-238.
- Mechanistic and functional insights into fatty acid activation in Mycobacterium tuberculosis. Nature Chem. Biol.; 2009, 5, 166-173.
- A novel tunnel in mycobacterial type III polyketide synthase reveals the structural basis for generating diverse metabolites. Nature Struct. Mol. Biol. ; 2004, 11, 894-900.
|
|